Data
You will be redirected to cenamweb.org in 15 second(s). The JINA-CEE website is not updated anymore. The archived website can still be reached through the CeNAM website.
Constraining Nuclear Level Densities for Insight into Ultradense Matter
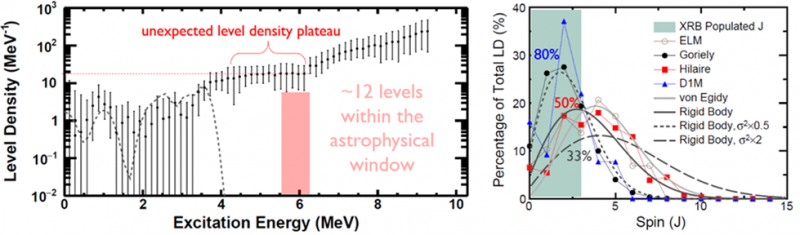
Type-I X-ray bursts on the surfaces of accreting neutron stars provide a unique window into the nature of matter at ultrahigh densities. One avenue for understanding dense matter properties could be X-ray burst model-observation comparisons. However, uncertain nuclear physics inputs limit the viability of this approach. A particularly key reaction is proton-capture gamma-emission, (p,γ), on the nucleus copper-59 (59Cu). Depending on the rate of this 59Cu(p,γ) reaction, energy generation during the X-ray burst may briefly stall, or not, impacting the shape of the burst's X-ray emission over time known as the light curve [1]. The abundance of mass 59 nuclides on the neutron star surface is also impacted, which can alter predictions of the outer neutron star thermal structure [2].
At present, 59Cu(p,γ) cannot be measured directly in the laboratory due to the short lifetime of 59Cu and challenges in radioactive ion beam production. Instead, theoretical calculations are required. A statistical approach known as the Hauser-Feshbach formalism has generally been used for this reaction. However, this assumes a substantial number of nuclear levels are available to be populated when a proton fuses with 59Cu to make zinc-60 (60Zn). Ohio University graduate student Doug Soltesz led the first measurement of the 60Zn nuclear level density. The measurement, performed at the Edwards Accelerator Laboratory at Ohio University, involved impinging a 10 MeV beam of helium-3 (3He) onto a nickel-58 (58Ni) target and detecting the spectrum of neutrons evaporated from this reaction. The neutron energy spectrum was related to the 60Zn level density, taking advantage of the fact that evaporation populates final levels statistically. More neutrons detected with a given energy means more levels are present at the corresponding excitation energy in 60Zn.
Our results show that the 60Zn level density is on the boundary of Hauser-Feshbach validity for the excitation energy region of interest for X-ray bursts (see Figure 1 left panel). This means that measurements of the quantum mechanical spin of the relevant levels, or just the spin distribution, are now necessary in order to determine how many astrophysically-relevant levels are present (see Figure 1 right panel). Our results also show an interesting plateau in the nuclear level density in the 60Zn excitation energy region of interest, contrary to the exponential increase expected from nuclear theory. This is the second observation of such a plateau, which is not presently understood theoretically.
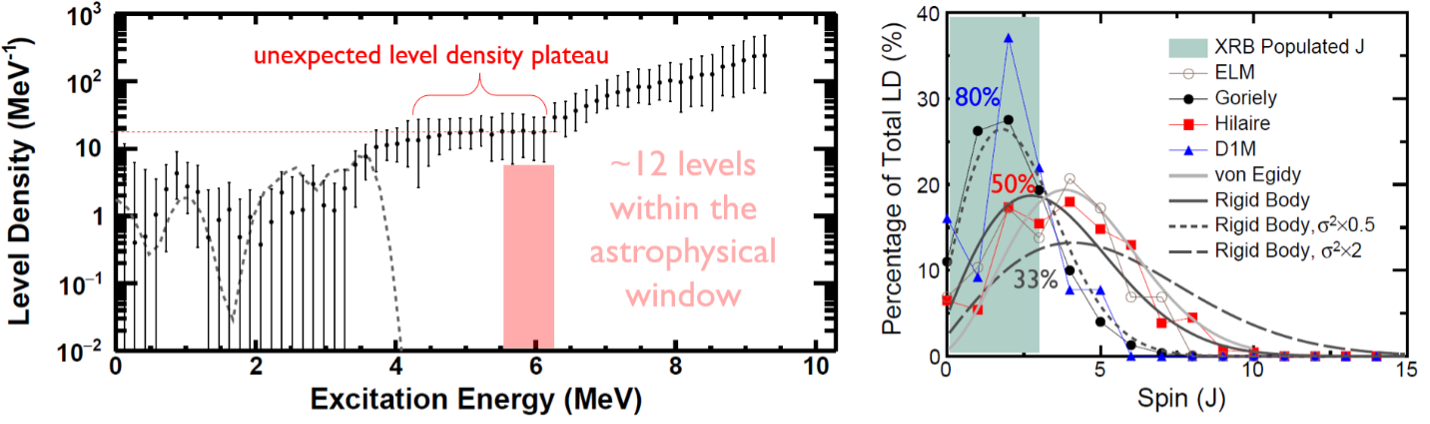
References:
[1] Meisel, Merz, & Medvid. Astrophys. J., 872, 84, 2019. (See March 2019 JINA Newsletter)
[2] Meisel & Deibel. Astrophys. J., 837, 73, 2017.